Overview
Background Information
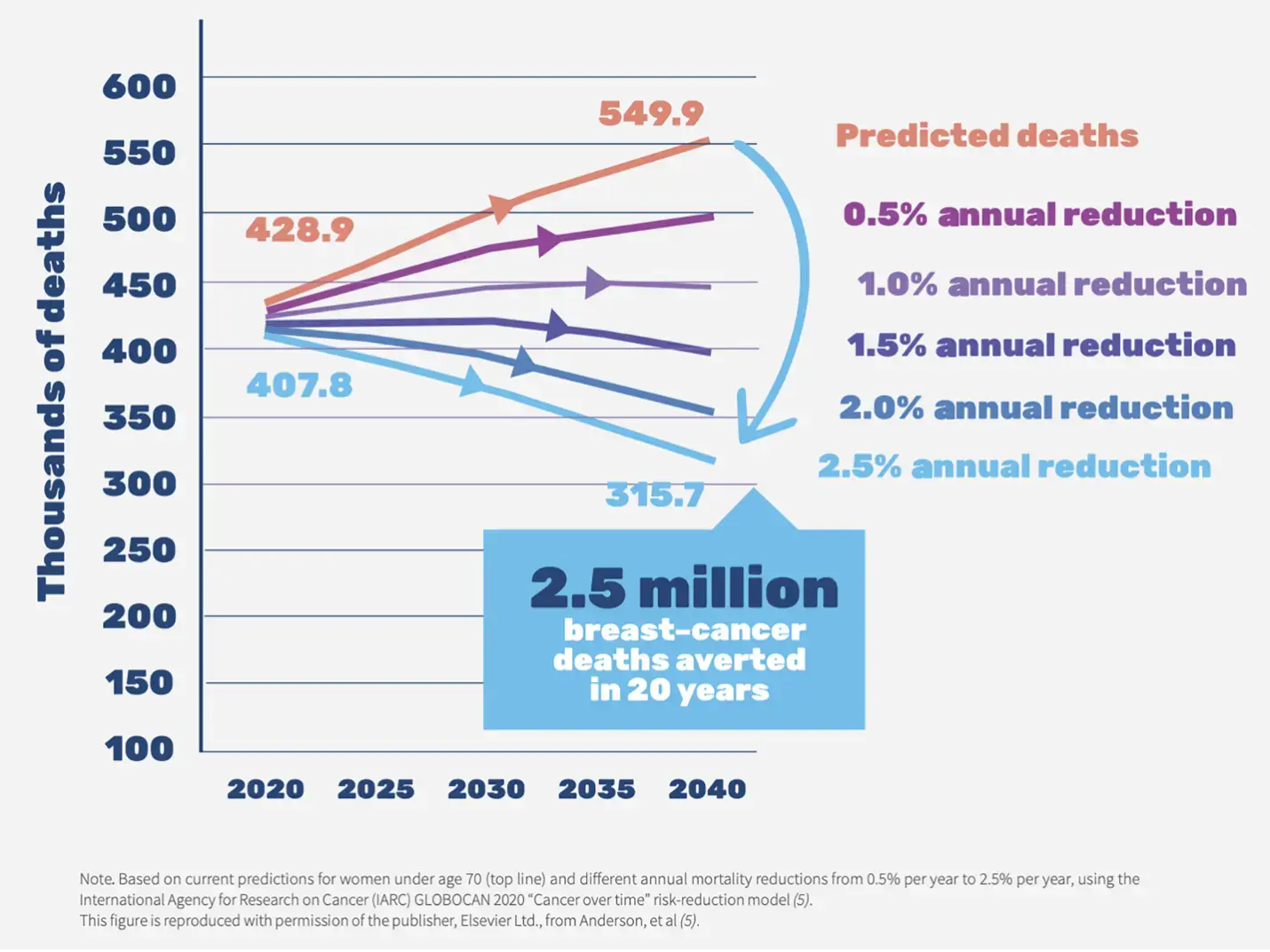
Figure 1. Breast cancer death rate
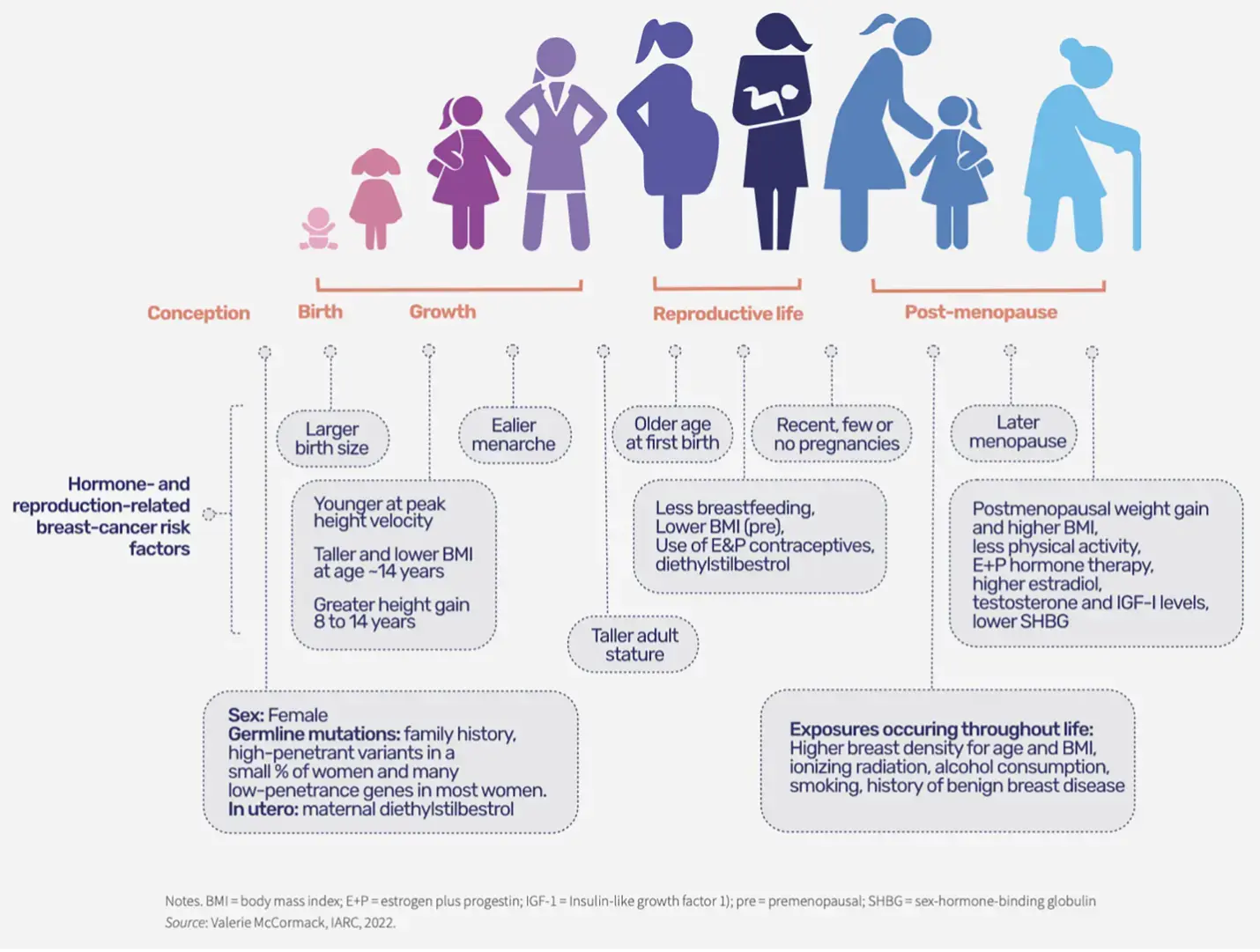
Figure 2. Breast cancer risk factors
Breast cancer is the second leading cause of cancer deaths in women (World Health Organization [WHO], 2022). According to data collected by World Health Organization, in 2022, 2.3 million women worldwide were diagnosed with breast cancer, and 670,000 died from the disease. Women in every country are susceptible to breast cancer at any age after puberty, with the incidence rate increasing as they age (American Cancer Society [ACS], 2023).
Breast cancer is a disease characterized by the uncontrolled growth of abnormal cells in the breast, leading to the formation of a tumor. The development of breast cancer is a multi-step process involving multiple cell types. Breast cancer prevention remains a challenge worldwide. Therefore, developing new breast cancer treatment strategies is crucial. In the last decade, great strides have been made in the understanding of breast cancer and in the development of therapeutic strategies. Treatment methods typically combine surgery, radiation therapy, and medication (Sun et al., 2017).
Up to now, researchers have identified a variety of genes associated with breast cancer. Mutations and aberrant amplification of oncogenes play a key role in tumorigenesis and progression. Among them, breast cancer-related genes 1 and 2 (BRCA1 and BRCA2) are two well-known breast cancer risk oncogenes (Gorodetska et al., 2019).
In recent decades, researchers have continuously developed tumor drugs, but curing breast cancer is still a huge challenge. The history of tumor drug development began in the 1940s with the synthesis of nitrogen mustard, the first clinically used tumor drug. This was followed by other early drugs like cyclophosphamide and 5-fluorouracil, which were among the first effective tumor drugs synthesized according to theory (Chau et al., 2019).
Introduction
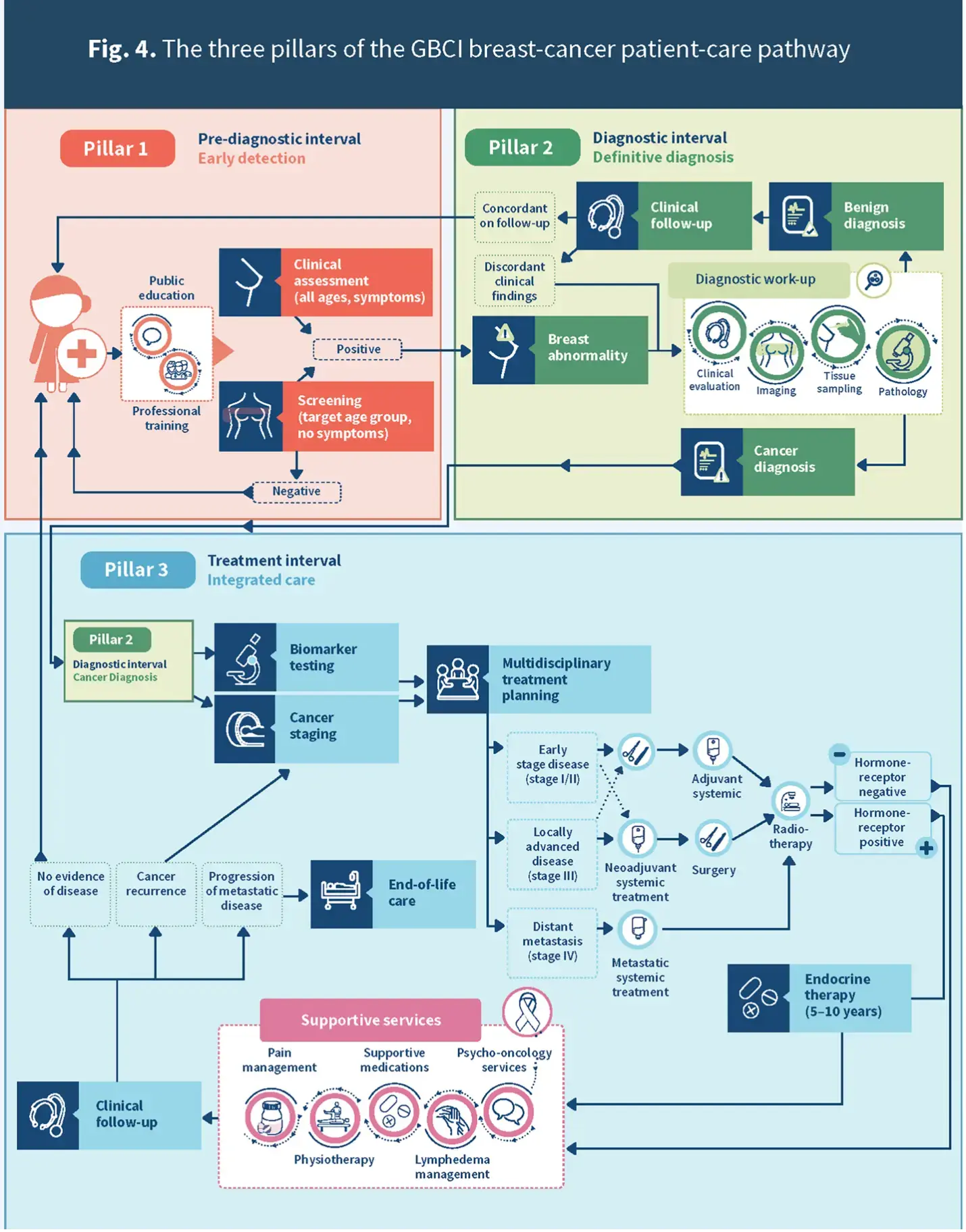
Figure 3. The three pillars of the GBCI breast cancer patient-care pathway
This project aims to develop a FRET-based biosensor to visualize intracellular ATP concentration, facilitating the efficient identification of new therapeutic agents for breast cancer by providing insights into apoptosis and physiological states.
Over the past two decades, the discovery and development of small molecule anticancer drugs have undergone a revolutionary transformation. Most notably, there has been a shift from a "one-size-fits-all" approach emphasizing cytotoxic chemotherapy to a personalized medicine strategy. There has been a greater focus on the discovery and development of molecularly targeted drugs that capitalize on the specific genetic addictions, dependencies and vulnerabilities of cancer cells.
This year, a family member of one of our team members was diagnosed with breast cancer. She informed us that a significant proportion of patients experience considerable anxiety following diagnosis, primarily due to concerns regarding the potential adverse effects of treatment and the risk of recurrence in the future. Our objective is to reduce the mortality rate of breast cancer by identifying drugs that can inhibit breast cancer cells through drug screening. Our team hopes to alleviate patients’ anxiety through this project.
Förster Resonance Energy Transfer (FRET) is a non-radiative energy transfer mechanism used to study intermolecular interactions and distance changes. lt relies on two main components: a donor fluorophore and an acceptor fluorophore. When the donor fluorophore absorbs a photon, it enters the excited state. If the donor is close enough to the acceptor fluorophore (usually in the range of 1-10 nanometers), the excitation energy of the donor can be transferred non-radiatively to the acceptor through dipole-dipole interactions. The acceptor fluorophore receives the energy, enters an excited state and subsequently emits photons. Therefore, this technique can be used to design fluorescent probes to detect the interaction of two molecules.
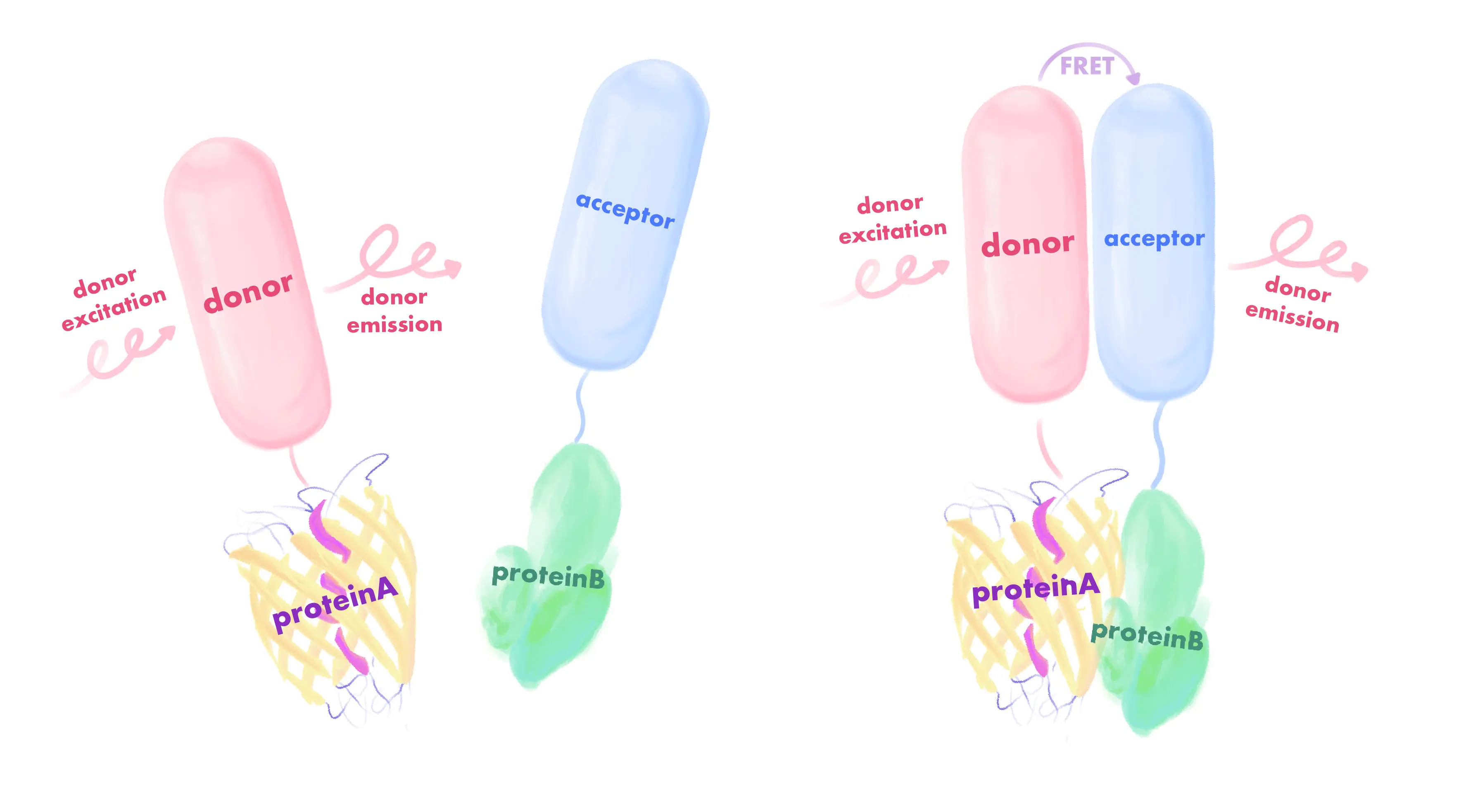
Figure 4. The FRET mechanism
Adenosine triphosphate (ATP) is a high-energy phosphate compound that can be interconverted with ADP to achieve energy storage and release in cells, thereby ensuring the energy supply for cellular life activities. Endogenous ATP is the most fundamental energy source for living cells. When cells die, ATP is rapidly hydrolyzed. Hence, the determination of endogenous ATP content can timely reflect the cell activity and the number of viable cells. ATP probes are molecules that bind to ATP and produce a fluorescent signal to detect ATP levels. The ATP fluorescent probe is the most widely used probe in the market. It can precisely detect the amount of ATP in the cell. However, its application in cell imaging is difficult, which limits its development. ATP-FRET probes utilize the energy transfer process of fluorescent protein pairs to detect ATP content. When two fluorescent proteins are in close proximity, fluorescence resonance energy transfer occurs, resulting in enhanced fluorescence emission from one fluorescent protein and diminished fluorescence emission from the other. By measuring the change in fluorescence signal, the ATP probe can indirectly measure ATP content. Therefore, it is of significance to enhance the ATP-FRET probe so that it can concurrently offer convenient and precise detection capabilities and be applicable for cell imaging. This design may improve the field of high-throughput drug screening.
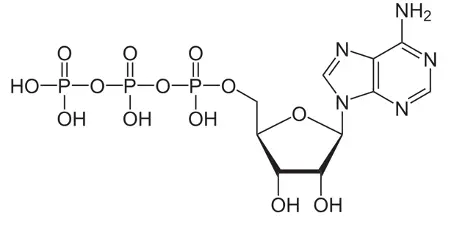
Figure 5. Adenosine triphosphate (ATP)
mBaoJin is a stable and bright fluorescent protein with high pH/chemical stability and rapid maturation. Compared to mNeonGreen, mBaoJin is approximately two times brighter intracellularly in neurons, which enhances the visualization of small cell processes. Under continuous wide-field illumination, mBaoJin photobleached four times slower than mNeonGreen and retains more than 50% of its initial fluorescence after 15 minutes of bleaching. Compared to eGFP and mNeonGreen, mBaoJin is significantly more photostable at low excitation intensities. At higher illumination powers (150-200 mW mm-2), mBaoJin is 2.5-4 times more photostable than mNeonGreen, making it suitable for applications requiring bright light. At the same time, mBaoJin is compatible with a wide range of super-resolution imaging modes, such as SIM and BaLM, and performs well at moderate and low excitation intensities, making it a versatile tool for researchers. In addition, it has been shown that the properties of mBaoJin could facilitate the development of single FP-based photostable biosensors, thereby extending the time scale of functional imaging.
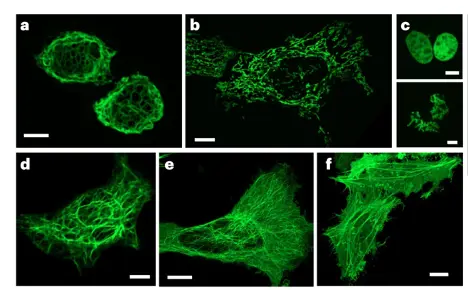
Figure 6. mBaoJin enables long-term super-resolution imaging of live HeLa cells. The images, presented in the sequence a–f, are confocal images of HeLa cells expressing mBaoJin fusions with vimentin (a), mitochondrial pre-sequence of human cytochrome c oxidase subunit VIII (b), H2B (bottom shows mitotic nuclei (c), keratin (d), α-tubulin (e) and β-actin (f) (n = 10, 9, 12, 4, 55 and 23 cells from four, three, two, two, four and two independent transfections, respectively)
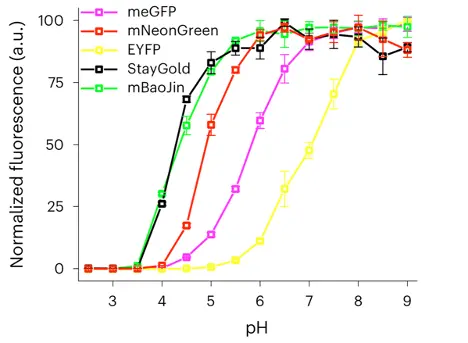
Figure 7. mBaoJin has high pH stability: fluorescence pH stability was evaluated in the presence of 300 mM NaCl (n = 3 technical replicates each; squares, mean; error bars, s.d.; fluorescence was normalised to the maximum intensity value of the corresponding FP)
Aquamarine has many advantages over other cyan fluorescent proteins (CFPs), especially compared to ECFP and mTurquoise. It has a quantum yield (QY) of 0.89, which is comparable to some of the top CFP mutants,indicating that the increased quantum yield shows that Aquamarine is able to emit fluorescence efficiently, making it ideal for sensitive imaging applications. Aquamarine has a high pH stability, with a pK1/2 of 3.3. Therefore, Aquamarine is able to maintain its fluorescence properties over a wider pH range than other CFPs. Because of its stability, Aquamarine can be used in situations where the pH may vary, like in some specialized cellular locations. With a fluorescence lifetime of approximately 4.12 ns, Aquamarine has a longer fluorescence lifetime than ECFP (2.55 ns). The longer fluorescence lifetime will enhance the signal of fluorescence lifetime imaging microscopy (FLIM), which will improve the resolution and sensitivity of detecting molecular interactions. The T65S mutation in Aquamarine reduces the occurrence of reversible photoswitching reactions. This reduction leads to more consistent and reliable imaging results, which minimizes the variability of the FRET signal, essential for accurate measurements in dynamic biological systems.
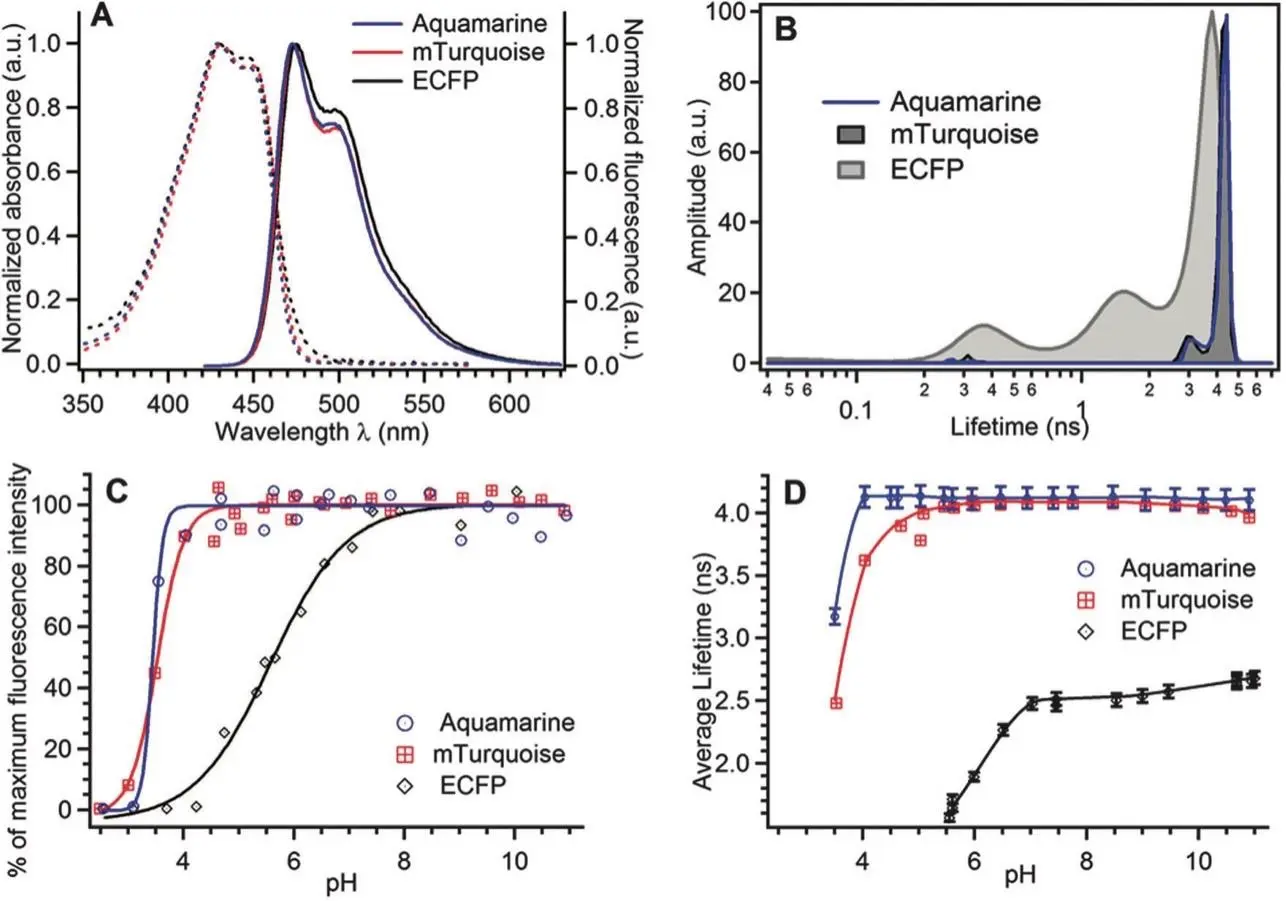
Figure 8. The photophysical properties of purified Aquamarine are hereby presented. (A) Normalised absorption (dashed lines) and emission (solid lines) spectra, with the chromophore band set at maximum. The emission spectra were recorded with excitation at a wavelength of 420 nm. (B) The fluorescence lifetime distributions at neutral pH were obtained from the MEM analysis of the fluorescence decay curves. (C) The pH dependence of the fluorescence intensity, excited at 420 nm and detected at 474 nm (Dl = 6 nm). The solid lines represent the optimal fits to a sigmoidal analytical model. The experimental data were normalised to 100% of the maximum value of their analytical fits. (D) The pH dependence of the average fluorescence lifetime was determined by integrating the lifetime distributions. The solid lines are intended solely for the purpose of providing guidance to the reader.
ScanCer
In ScanCer, we refer to previous researchers' use of FRET technology for comparing ATP levels in the mitochondrial matrix of HeLa cells with those in the cytoplasm and nucleus, and use FRET as the technical basis to improve an efficient ATP detection probe for high-throughput drug screening and fluorescence imaging. Using the sensor that we have developed, we screened breast cancer medicines and conducted functional validation.
In this study, we employed an innovative biosensing technology, the pCDH-AεB sensor. This sensor features a unique design that integrates the fluorescent protein Aquamarine with an optimized yellow fluorescent protein (mBaoJin), embedded within the epsilon domain of ATP synthase derived from Bacillus subtilis (ID AFQ603.1). Notably, the epsilon domain plays a crucial role in ATP binding; upon ATP interaction, it undergoes conformational changes that subsequently influence FRET efficiency. Under natural conditions, without drug interference, the distance between Aquamarine and mBaoJin remains relatively large, resulting in a weak FRET effect. However, when cells are treated with anticancer drugs, fluctuations in intracellular ATP concentration occur, leading to a conformational adjustment in the epsilon domain that reduces the distance between the two proteins and significantly enhances the FRET effect.
During the drug screening experiments, we cultured host cells containing the pCDH-AεB biosensing elements in a 96-well plate, simultaneously introducing various potential anticancer drugs into the co-culture system. Utilizing a high-content fluorescence detection system, we precisely measured the fluorescence emission ratio between Aquamarine and mBaoJin, enabling dynamic tracking of fluctuations in intracellular ATP concentration following drug treatment. Specifically, the drug-induced changes in ATP levels triggered alterations in the spatial configuration of the epsilon domain, thereby modulating the FRET effect, which can be visually reflected by subtle changes in mBaoJin fluorescence intensity.
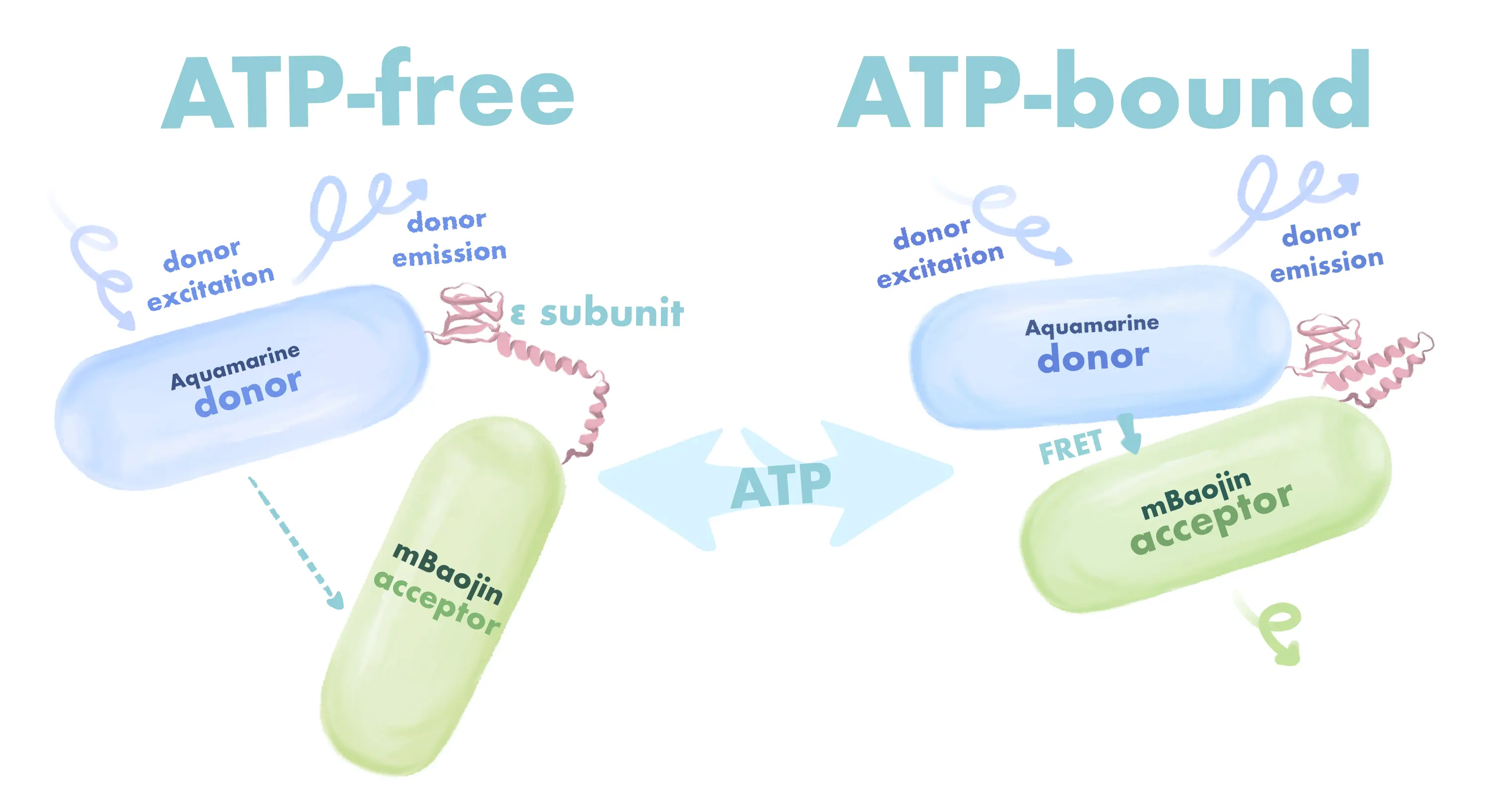
Figure 9. The working mechanism of our sensor
In the data analysis phase, we compared the differences in mBaoJin fluorescence intensity between the treatment group (with drug intervention) and the control group (without drug treatment) to evaluate the changes in intracellular ATP concentration induced by the drugs. This strategy effectively identifies potential anticancer compounds that can significantly regulate ATP levels.
Additionally, we conducted supplementary validation experiments for the promising drug candidates identified in the initial screening, aimed at confirming their efficacy and enhancing their pharmacological properties. This validation process not only established the effectiveness of the pCDH-AεB biosensor in the drug screening phase but also contributed valuable research data for future drug development and cancer therapy.
In summary, our research findings highlight the promising applications of the pCDH-AεB biosensing technology in the field of high-throughput drug screening, particularly emphasizing its capability to accurately and efficiently assess changes in intracellular ATP concentration triggered by anticancer drugs. Through this FRET-based biosensing approach, we provide an innovative strategy for exploring and developing novel anticancer therapeutics.
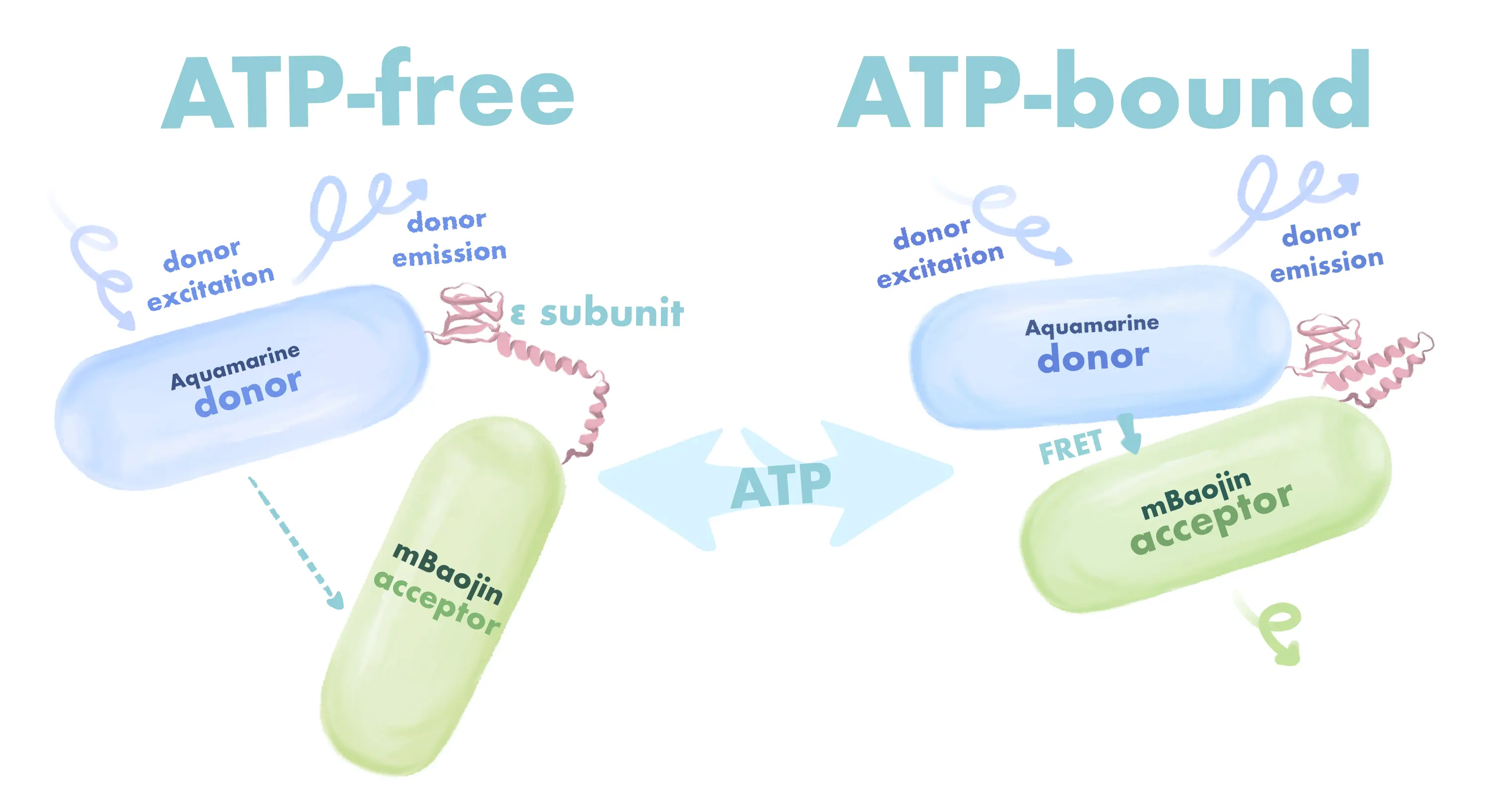
References
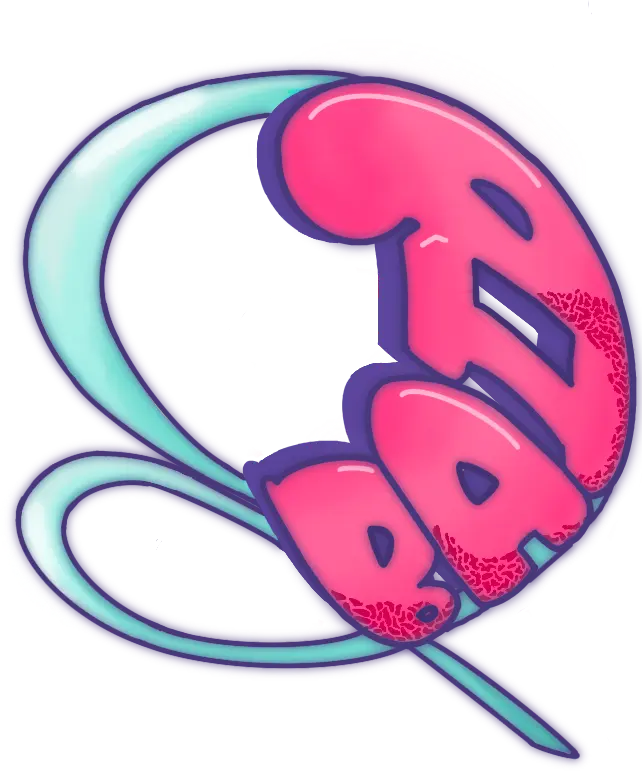
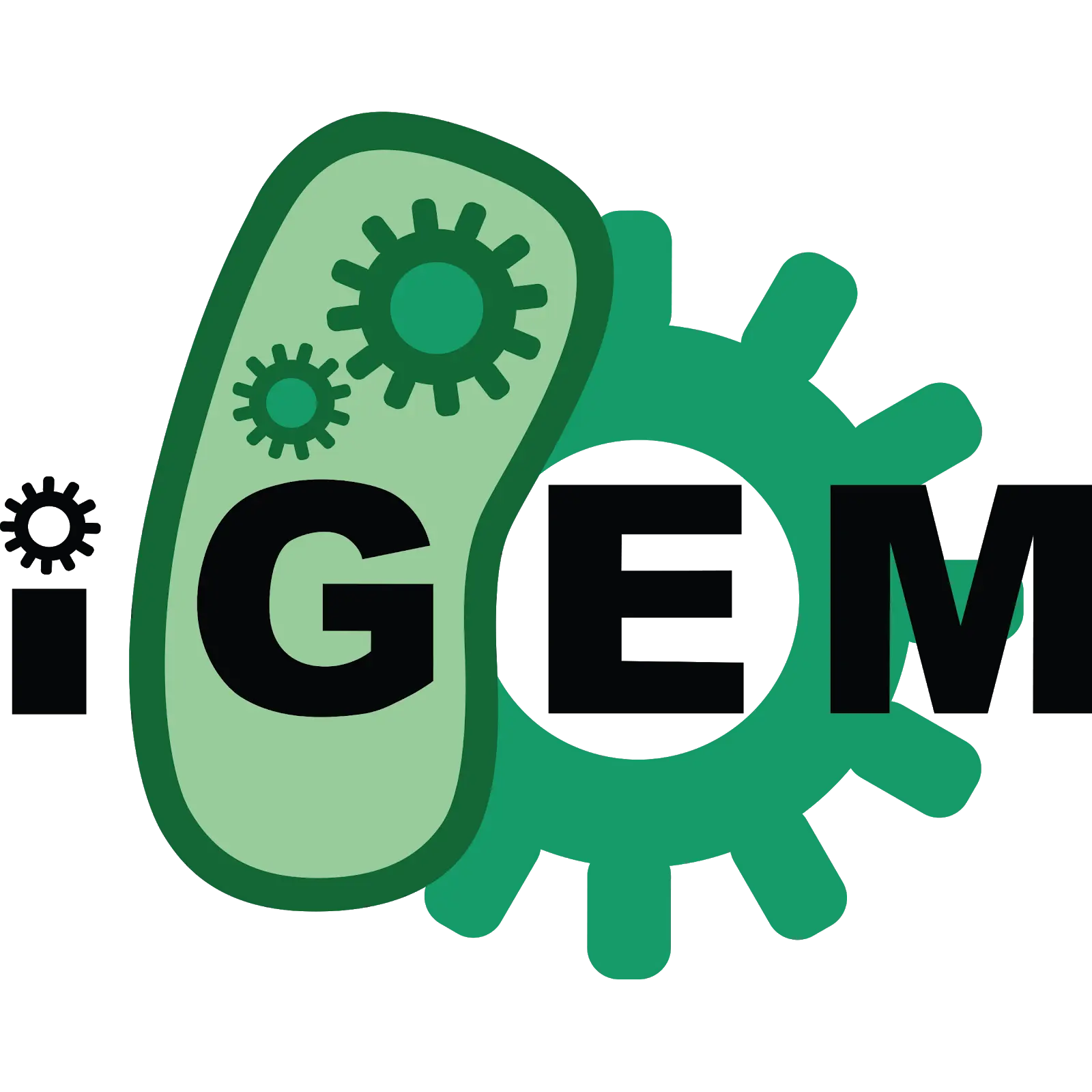